Impact Of Wind-Induced Vibrations On Aluminum Conductor Fatigue: A Novel Testing Approach
DOI:
https://doi.org/10.14295/vetor.v34i2.18318Keywords:
Aluminium Alloy Conductor, Eolian – vibration, Self-damping, Transmission lineAbstract
In transmission lines, wind-induced vibrations, like high-frequency wind vibrations and low-frequency galloping phenomenon, can cause fatigue damage and failure, particularly in aluminum conductors, which have greater flexibility. Wind loads are variable forces applied by the wind to structures, including conductors. These dynamic wind loads vary with weather and altitude where the conductors are located, causing mechanical dissipation energy due to wire friction, particularly in contact zones. To ensure stability and safety, these loads and the damping effect must be taken into account when planning electrical transmission systems. The aim of this study is to investigate the impact of wind loads on the conductor's self-damping characteristics using a new approach to self-damping testing. Indeed, a self-damping test was carried out at the University of Brasilia's fatigue laboratory on 838 MCM AAAC 1120. The test bench was operated at frequencies ranging from 15.25 to 35.55 Hz, with the catenary parameter H/w = 2143 m, representing the ratio between the initial horizontal tensile load (H) and the conductor weight (w) per unit length. The power dissipated per unit length was calculated using the power method. It uses a methodology that allows the dissipation of mechanical energy to be measured accurately. The analysis revealed that higher frequencies lead to greater energy dissipation. Higher normalized amplitude with respect to the conductor diameter (Y/D ratio) lead to greater dissipation at the same frequency. The increase follows a power-law behavior, which is typical of vibration systems where the dissipated energy depends on frequency and amplitude.
Downloads
References
J.-P. Hou, Q. Wang, H.-J. Yang, X.-M. Wu, C.-H. Li, Z.-F. Zhang, and X.-W. Li, “Fatigue and Fracture behavior of a Cold-Drawn Commercially pure aluminum wire,” Materials, vol. 9, no. 9, p. 764, 2016. Available at: https://doi.org/10.3390/ma9090764
J. A. Redford, M. Gueguin, M.C. Nguyen, H.-P. Lieurade, C. Yang, F. Hafid, and J.-M. Ghidaglia, “Calibration of a numerical prediction methodology for fretting-fatigue crack initiation in overhead power lines,” International Journal of Fatigue, vol. 124, pp. 400–410, 2019. Available at: https://doi.org/10.1016/j.ijfatigue.2019.03.009
A. A. Fadel, D. Rosa, L. B. Murça, J. L. A. Fereira, and J. A. Araújo, “Effect of high mean tensile stress on the fretting fatigue life of an Ibis steel reinforced aluminium conductor,” International Journal of Fatigue, vol. 42, pp. 24–34, 2012. Available at: https://doi.org/10.1016/j.ijfatigue.2011.03.007
C. Poon, S. U. Mhurchadha, R. A. Barrett, and S. B. Leen, “Three-dimensional representative modelling for fretting wear and fatigue of submarine power cable conductors,” International Journal of Fatigue, vol. 184, 2024. Available at: https://doi.org/10.1016/j.ijfatigue.2024.108302
P. H. C. Rocha, S. Langlois, S. Lalonde, J. A. Araújo, and F. C. Castro, “Influence of 1350 and 6201 aluminum alloys on the fatigue life of overhead conductors – A finite element analysis,” Tribology International, vol. 186, 2023. Available at: https://doi.org/10.1016/j.triboint.2023.108661
R. B. Kalombo, J. M. G. Martínez, J. L. A. Ferreira, C. R. M. Da Silva, and J. A. Araújo, “Comparative Fatigue Resistance of Overhead Conductors Made of Aluminium and Aluminium Alloy: Tests and Analysis,” in Procedia Engineering, vol. 133, 2015, pp. 223–232. Available at: https://doi.org/10.1016/j.proeng.2015.12.662
M. A. Garcia, R. K. Badibanga, J. A. Araújo, J. L. A. Ferreira, and C. R. Moreira da Silva, “Fretting fatigue tests on 6201-T81 aluminum alloy conductor wires at room temperature and 75 °C,” Wear, vol. 548–549, p. 205321, 2024. Available at: https://doi.org/10.1016/j.wear.2024.205321
A. V. Silva, M. A. Costa, H. Ahn, and A. L. M. Lopes, “Performance benchmarking models for electricity transmission regulation: Caveats concerning the Brazilian case,” Utilities Policy, vol. 60, p. 100960, 2019. Available at: https://doi.org/10.1016/j.jup.2019.100960
M. Shahbaz, G. Gozgor, and S. Hammoudeh, “Human capital and export diversification as new determinants of energy demand in the United States,” Energy Economics, vol. 78, pp. 335-349, 2019. Available at: https://doi.org/10.1016/j.eneco.2018.11.016
S. K. Teegala and S. K. Singal, “Optimal costing of overhead power transmission lines using genetic algorithms”, International Journal of Electrical Power and Energy Systems, vol. 83, pp. 298-308, 2016. Available at: https://doi.org/10.1016/j.ijepes.2016.04.031
N. Kannan and D. Vakeesan, “Solar energy for future world: - A review,” Renewable and Sustainable Energy Reviews, vol. 62, pp. 1092-1105, 2016. Available at: https://doi.org/10.1016/j.rser.2016.05.022
C. A. Cimini and B. Q. A. Fonseca, “Temperature profile of progressive damaged overhead electrical conductors,” International Journal of Electrical Power and Energy Systems, vol. 49, no. 1, pp. 280-286, 2013. Available at: https://doi.org/10.1016/j.ijepes.2012.12.015
T. Ishihara and T. Li, “Numerical study on suppression of vortex-induced vibration of circular cylinder by helical wires,” Journal of Wind Engineering and Industrial Aerodynamics, vol. 197, p. 104081, 2020. Available at: https://doi.org/10.1016/j.jweia.2019.104081
F. Luo, C. Gao, and W. Zhang, “The key to suppress vortex-induced vibration: Stability of the structural mode,” Journal of Fluids and Structures, vol. 113, p. 103692, 2022. Available at: https://doi.org/10.1016/j.jfluidstructs.2022.103692
W. L. Chen, Q. Q. Zhang, H. Li, and H. Hu, “An experimental investigation on vortex induced vibration of a flexible inclined cable under a shear flow,” Journal of Fluids and Structures, vol. 54, pp. 297–311, 2015. Available at: https://doi.org/10.1016/j.jfluidstructs.2014.11.007
H. Y. Guo and M. Lou, “Effect of internal flow on vortex-induced vibration of risers,” Journal of Fluids and Structures, vol. 24, no. 4, pp. 496–504, 2008. Available at: https://doi.org/10.1016/j.jfluidstructs.2007.10.002
P. W. Bearman, “Vortex shedding from oscillating bluff bodies,” Annual review of fluid mechanics, vol. 16, pp. 195-222, 1984. Available at: https://doi.org/10.1146/annurev.fl.16.010184.001211
T. Sarpkaya, “Vortex-Induced Oscillations a Selective Review,” Journal of Applied Mechanics, Transactions ASME, vol. 46, no. 2, pp. 241-258, 1979. Available at: https://doi.org/10.1115/1.3424537
G. Diana, M. Falco, A. Cigada, and A. Manenti, “On the measurement of overhead transmission lines conductor self-damping,” IEEE Transactions on Power Delivery, vol. 15, no. 1, pp. 285-292, 2000. Available at: https://doi.org/10.1109/61.847264
F. Foti and L. Martinelli, “An enhanced unified model for the self-damping of stranded cables under aeolian vibrations,” Journal of Wind Engineering and Industrial Aerodynamics, vol. 182, pp. 72-86, 2018. Available at: https://doi.org/10.1016/j.jweia.2018.09.005
T. Barbosa de Miranda, R. K. Badibanga, J. A. Araujo, and J. Ferreira, “Self-damping of conductors AAC, AAAC and ACSR with respect of H/W parameter”, in 25th ABCM International Congress of Mechanical Engineering, Uberlândia, Brazil: ABCM, 2019. Available at: https://doi.org/10.26678/abcm.cobem2019.cob2019-1666
IEC 62567, Overhead lines: methods for testing self-damping characteristics of conductors, 1st Edition, International Electrotechnical Commission and Technical Committee 7: Overhead electrical conductors. 2013.
IEE Std 664TM-1993 (R2007), “IEEE guide for laboratory measurement of the power dissipation characteristics of aeolian vibration dampers for single conductors,” in IEEE Std 664-1993. pp. 1-24, 1993. Available at: https://doi.org/10.1109/IEEESTD.1993.119669
K. W. Barber and K. J. Callaghan, “Improved overhead line conductors using Aluminium Alloy 1120,” in Proceedings of the IEEE Power Engineering Society Transmission and Distribution Conference, Chicago, IL, USA: IEEE, 1994, pp. 528-534. Available at: https://doi.org/10.1109/TDC.1994.328420
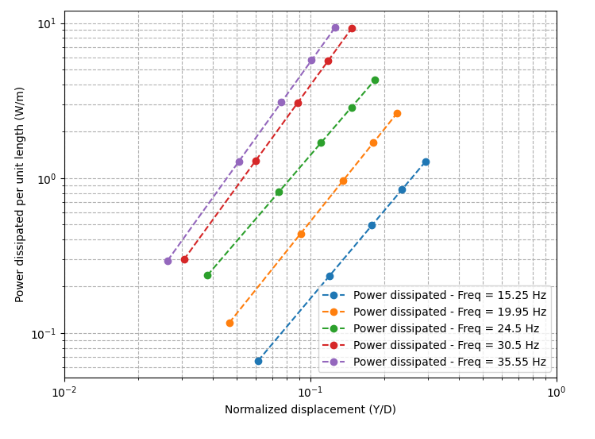